‘Quantum Light’
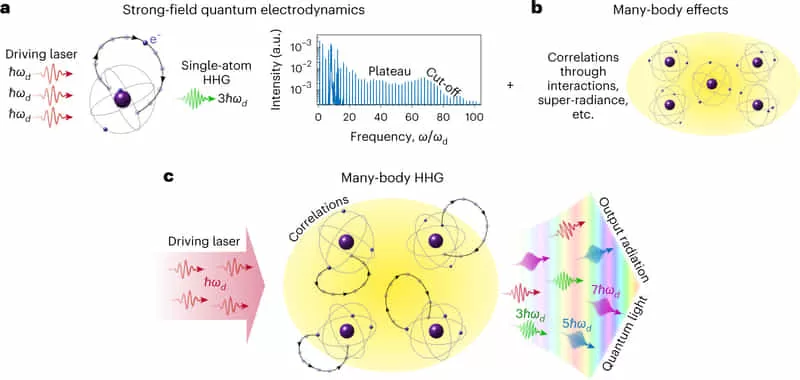
Quantum theory of light emission by strongly driven many-body atomic systems.a, HHG can be understood as a single-particle, strong-field three-step process: (1) an intense drive laser tears off an electron from the atom, (2) the electron is accelerated by the electric field and (3) the electron recombines with the atom, converting its energy into an energetic photon at higher harmonics. The spectrum features are peaks at the odd harmonics, a characteristic plateau and a cut-off. b, The many-body correlations among the atoms can arise from spontaneous collective emission (super-radiance) or inter-atomic interactions. c, Our theory marries the description of many-body effects with that of strong-field physics, giving access to unique quantum properties of the emitted light.
Strongly driven systems of emitters offer an attractive source of light over broad spectral ranges up to the X‑ray region. A key limitation of these systems is that the light they emit is mostly classical. We overcome this constraint by building a quantum-optical theory of strongly driven many-body systems, showing that the presence of correlations among the emitters creates emission of non-classical many-photon states of light. We consider the example of high-harmonic generation, by which a strongly driven system emits photons at integer multiples of the drive frequency. In the conventional case of uncorrelated emitters, the harmonics are in an almost perfectly multi-mode coherent state lacking any correlation between harmonics. By contrast, a correlation of the emitters before the strong drive is converted into non-classical features of the output light, including doubly peaked photon statistics, ring-shaped Wigner functions and correlations between harmonics. We propose schemes for implementing these concepts, creating the correlations between emitters via an interaction between them or their joint interaction with the background electromagnetic field. Our work paves the way towards the engineering of novel states of light over a broadband spectrum and suggests high-harmonic generation as a tool for characterizing correlations in many-body systems with attosecond temporal resolution.
The creation and control of many-photon quantum states of light are important problems with applications across the natural sciences. Realizations of squeezed quantum light states open new avenues in spectroscopy and metrology, providing novel information on samples1 and enabling highly sensitive measurements beyond classical noise limits (for example, in the detection of gravitational waves2,3). At the same time, encoding quantum information on the quantum state of light facilitates applications in quantum computing, simulation and communication4. Several pioneering investigations have demonstrated a range of many-photon quantum states of light such as squeezed light2,3,5 – 7, bright squeezed vacuum8 – 11, displaced Fock states12, Schrödinger kitten13,14 and cat states15,16, subtracted squeezed states17 and others18. Many of the established techniques for generating quantum light at optical frequencies rely on materials with a non-linear optical response. Such non-linear materials can be typically described using a ‘perturbative’ non-linear response, where the induced polarization is, for example, quadratic or cubic in the applied electric field. At the other extreme of non-linear optics are ‘non-perturbative’ or ‘strong-field’ effects such as high-harmonic generation (HHG), in which a very intense optical pulse creates radiation at very high frequencies, even beyond hundred fold the frequency of the drive19,20. As such, HHG is an attractive source of ultra-short pulses of high-frequency light. The potential of HHG for the generation of non-classical high-frequency light has, however, remained largely unexpressed.
Keine Kommentare